Around the world, the demand for information anywhere and anytime continues to grow in commercial and military applications. While the previous decade focused on mobile communication, the current decade is focusing on mobile broadband. Today’s theme is “access to everything, everywhere,” and the growth in users and devices is driving tremendous growth in the demand for broadband data.
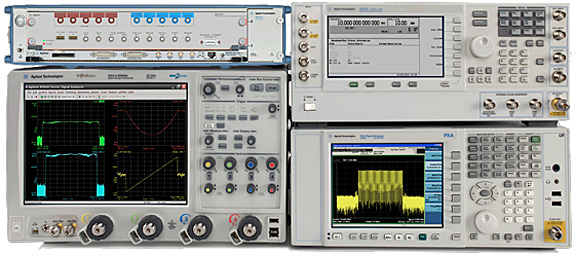
Figure 1: This four-instrument test setup enables testing of both receivers and transmitters.
To support this growth, satellites are a critical part of the infrastructure. One way to ensure an increase in broadband access is to place new satellites in orbit, and industry watchers are forecasting an increase in launches. Putting a new bird in the air presents numerous challenges. In particular, the need to ensure interoperability between new and existing communication links. Such links may be required between terrestrial and space-based communications or between satellites and multiple types of military radios.
As an alternative to placing more satellites in orbit, the capacity of existing satellites can be enhanced. New modulation techniques make it possible to pack more information into the existing transmission bandwidth, and this enables higher data rates and thereby an expansion of overall system capacity. As an example, using 16QAM and 1 GSymbol/s can provide a 4Gb/s data rate.
For new or existing satellites, a link involves the transmission of a signal from an Earth station to a satellite. The satellite receives the signal, amplifies it and sends it back Earthward. Stress testing of these links requires long data transmissions that include changes to various signal parameters.
All of this presents significant challenges in the creation of the test signals that are needed to exercise satellite receivers. Today, few instruments are capable of satisfying the need for high-quality signals, on-the-fly changes and long playback times.
Outlining The Desired Test Setup
On the receiver side, the first item in the test setup is an arbitrary waveform generator (AWG), which can simulate the necessary modulated signals. Next is an upconverter, which translates the modulated signal upward to the required RF frequency.
Measurements on the transmitter side benefit from two devices: A signal or spectrum analyzer and a wideband oscilloscope. Either of these may be enhanced with vector signal analysis (VSA) software that provides the necessary demodulation capabilities and essential measurements such as error vector magnitude (EVM). Figure 1 shows an example setup that can be used to test satellite receivers and transmitters.
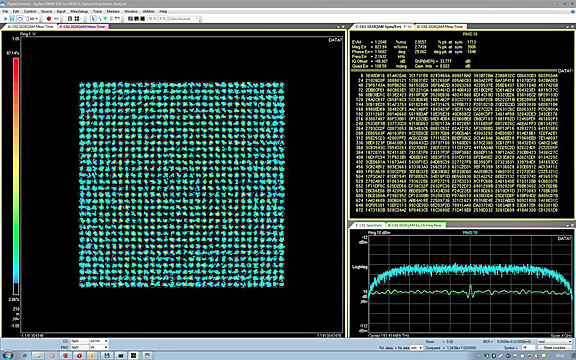
Figure 2. This trace shows a 1024QAM, 3Gbd optical transmission generated by the Agilent M8190A.
Understanding Typical Signal Types
A new generation of high-speed AWGs provides several advantages in satellite testing. In addition to being highly versatile, current-generation AWGs continue to move upward in bandwidth while also providing good signal quality due to high vertical resolution.
This suggests an initial question: Is an arbitrary waveform generator a good choice for stressing a satellite link to its limits? In the satellite system, the main goal is to squeeze as much data as possible into the spectrum. This calls for high spectral efficiency, which measures the throughput in an assigned bandwidth expressed as bits- per-second per hertz. One technique that provides high spectral efficiency is quadrature amplitude modulation (QAM, because it uses combinations of amplitude and phase, QAM can transmit more bits per symbol.
During satellite testing, the use of QAM requires ongoing changes to amplitude and phase, especially when characterizing sensitivity. To support such tests, the AWG needs to allow a high order of modulation, and QAM1024 is possible with new-generation instruments (please see Figure 2 on the next page). A QAM1024 signal can be represented in 10 bits/symbol. The 1024QAM measurement highlights the value of an AWG with high signal quality. For example, precise positioning of different points is critical for high-order modulations. On the receiving side, demodulation becomes difficult in the presence of noise, which is always present in real-world wireless environments.
Another useful modulation technique is orthogonal frequency domain multiplexing (OFDM), which is widely used in communication systems. A transmission channel is split into several subcarriers, and combining subcarriers with standard modulations provides both a high data rate and little or no crosstalk. As subcarrier frequency and spacing are orthogonal to each other, the spectra don’t interfere with one another.
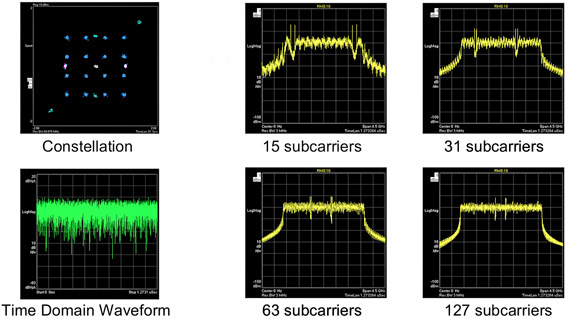
Figure 3: This OFDM signal, which has QAM16 modulation, a different subcarrier and two pilots, was generated by an Agilent M8190A AWG.
By adding subcarriers, the signals become flatter—and a larger number of subcarriers brings the signal closer to being ideal (please see Figure 3 on the next page). Because OFDM is sensitive to variations in carrier frequency, it transmits pilot carriers along with the subcarriers for synchronization at the receiver.
OFDM has a high spectral efficiency due to the close spacing of the individual carriers. The method is robust in the presence of interference and noise because the loss of an individual carrier is not fatal to the entire transmission. Any loss can be recovered through its error-correction algorithm.
When both the transmitter and receiver are moving, high reliability is needed. In such cases, OFDM seems to be a perfect mechanism because it mitigates the problem of multipath propagation.
Last but not least, the complexity of OFDM makes unauthorized demodulation virtually impossible. The reason: OFDM signals can contain different encoding, mapping types, preambles, payloads and numbers of subcarriers.
The use of OFDM does have disadvantages. For example, an OFDM signal has a high peak power ratio, which requires linear amplification and test instruments that have high dynamic range.
Taking A Closer Look At The AWG
To generate the required signals, an arbitrary waveform generator must have sufficient resolution to produce modulated signals with high dynamic range. Playtime is another important consideration. Testing a receiver within one transmission link can be done either within a short test or with a long playtime. In the past, the answer was adding more memory to the instrument. In truth, though, there is never enough memory.
This is one reason why many high-speed AWGs provide a huge amount of memory; however, the actual possible playtime depends on several factors including the nature of the signal itself. For example, assume a 50GSa/s signal and 16GSa of built-in memory: this equates to just 320ms of playtime. Unfortunately, required measurement times are typically minutes in duration. Adding sequencing capabilities can extend the available playtime, and a sequencer that supports repetitive signals can achieve an essentially endless playback time.
What if the test scenario requires unique signals in different scenarios? Standard AWGs certainly have limitations. A generated signal is downloaded into AWG memory and then sent to the DAC output. Whenever the signal must be changed, the measurement must be stopped. This is a crucial point if the test scenario requires a data stream that is both constant and variable. One reason: stopping the measurement also means stopping the transmission link. As a result, it isn’t possible to produce the continuous real-life signal scenarios needed to perform long stress tests.
Agilent recently added digital up-conversion to its M8190A, which overcomes the typical AWG limitation. Now a test engineer can make on-the-fly changes to parameters such as amplitude, frequency and phase without restarting the AWG. Thus, permutations of an existing signal are possible without stopping the measurement or the transmission link.
This is possible because signal parameters are stored independently of the waveform itself. As a result, they can be changed by the sequencer during runtime. Specific to satellite testing, this enables accurate testing of channels and transmitters by ensuring that amplitude and phase won’t drift or degrade over time.
Playback time is also a very important topic for satellite applications. Test instruments provide a sequencer, which enables long play times for repetitive waveforms. The M8190A supports streaming to enable continuous or ongoing output of changing waveforms. The source can be an uninterrupted data stream from a disk or an algorithm, or it can be data downloaded to memory from an external digitizer or oscilloscope while the AWG outputs the signal.
For some tests, simply outputting an ongoing and changing waveform might be sufficient. However, in other cases it is important to have control over the timing. This can be used to help precisely pinpoint the cause of a problem. In other cases, it may be useful to react dynamically to real-time events and change the waveform as soon as an event occurs. The M8190A provides streaming modes for deterministic timing as well as support for dynamic signal scenarios.
Enhancements Required To Address New Technologies
The goal of packing more data into existing satellite bandwidth can be achieved with complex modulated signals. Addressing these new technologies requires enhancements to satellite hardware and the instruments used to test it. AWGs can keep pace with the new requirements if they continue to advance in signal quality, frequency and memory, and include or support functionality that extends playtime.
About the author
Beate i is the Product Manager, Pulse Pattern Generators, Digital Test Division within the Electronic Measurements Group. She designs and implements marketing strategies for the most comprehensive pulse and data generator product portfolio for Agilent Technologies’ Digital Verification Solutions Division. Beate is responsible for the marketing activities along the entire product life cycle. Beate joined Agilent Technologies (formerly Hewlett-Packard) in 1989 as a consultant in the computer business. During her 16 years with Agilent, she held various positions within marketing including strategic product and business planning within the Network and System Management Division, sales development for a start-up business within Agilent, and is also the Marketing Manager for an OEM Business that is focused on customer relationship management. Beate was born in Paderborn, Germany in 1965 and holds a degree in Business Computer Science.
* * * * * * * * *
High Performance Arbitrary Waveform Generators
Agilent’s high performance arbitrary waveform generators use low noise clocks which provide unmatched performance and high-quality signal generation to achieve superior fidelity.
Whether you need benchtop, system ready AXIe, LXI or PXI, Agilent’s high performance arbitrary waveform generators offer clean, complex and real-world signals. Advanced sequencing optimizes memory to simulate long duration events. Agilent Arbitrary Waveform Generators help you achieve world class signal generation in many applications
Aerospace applications in satellite, radar, Electronic Warfare (EW)—push system designs farther with highly realistic signals. Agilent AWGs offer higher resolution to discriminate close in targets and low level signals
Communications applications in Satellite, UWB, IEEE 802.11ad/ac—Build a strong foundation for highly reliable satellite communication. Agilent AWGs offer high data rates, less signal uncertainty and interoperability
Serial bus Standards—Generate multi-level signals and reproduce analog imperfections with programmable ISI and jitter up to 3Gb/s
“Get it right the first time” takes on a larger meaning with satellites going into Earth´s orbit or traveling deep into space. From guidance systems to satellite payloads to microwave communications, design and validation tools from Agilent provide greater assurance that your satellite and its subsystems will work the first time, every time for the duration of the mission.