Over the summer, members of the global smallsat community gathered for a smallsat conference in Logan, Utah. The attendees, representing 45 countries and 1,100 organizations, took part in discussing this year’s conference theme, “Driving a Revolution.”
How does this theme describe the current state of the smallsat industry? To understand, it is worth looking back at how other industries have revolutionized our world today. Innovative advancements in technology, standardization and interoperability, and automation were the foundations upon which the automotive, aviation, and computer industries were built.
How will the current Smallsat Revolution be successful? Similar to past technological revolutions, there needs to be synergy between today’s emerging technologies and the tools that have stood the test of time. Advancements in high-performance, low-power processing and primary/secondary/tertiary launch must come together with standards and interoperability to fuel new and innovative space missions that were not possible even ten years ago.
The continuous growth of the industry is evident through the examination of many indicators. Estimates of the current worldwide smallsat market from SpaceWorks Enterprises, Inc.’s 2019 Nano/Microsatellite Market Forecast (9th Edition) predicted almost 300 nano/micro-satellite launches in 2019, representing a 17 percent increase over the 2018 numbers.
What has fueled this continued growth? And, more importantly, where is the market going? To answer these questions, it is prudent to reflect on the past and the innovations that have brought smallsats to where they are today.
A History of Smallsats
During the 1950’s and 1960’s, spacecraft designs were restricted by launch vehicle payload mass and on-board computer processing performance ceilings.
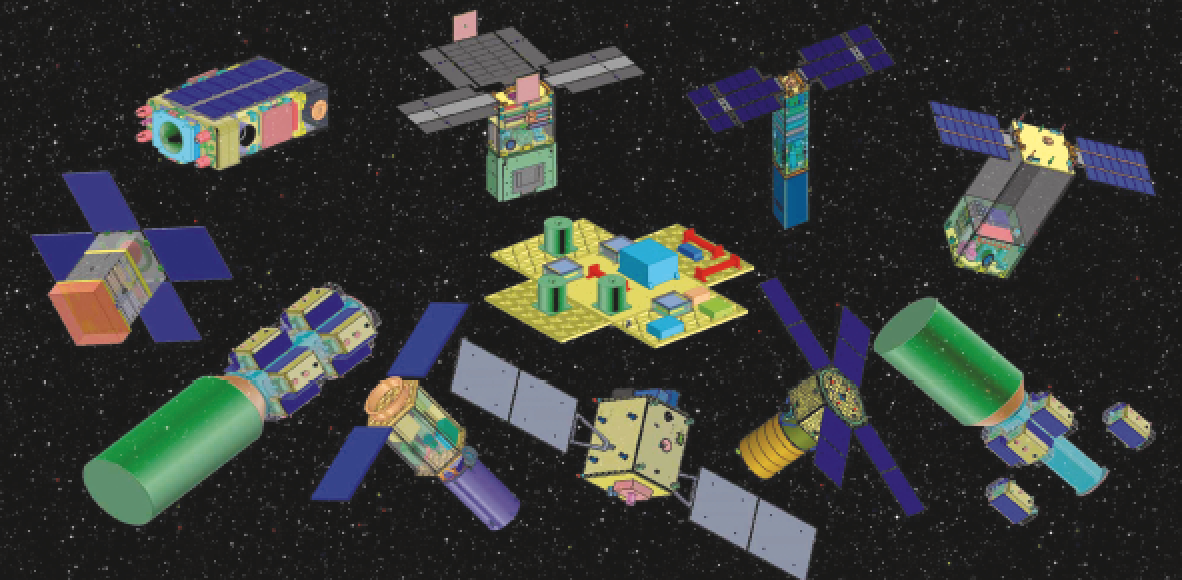
The limitations of launch vehicle throw-weight at the time made Smallsats the original satellite class, while low computing power limited them to technology development and experimental units.
The personal computer revolution of the 1970’s and 1980’s introduced higher-processing capability into spacecraft bus and mission designs. Though access to space remained expensive due to limited launch availability, increased functional and performance capabilities enabled significantly more capable spacecraft to be built.
These high performance, operational satellites needed to achieve long mission life, which required reliability.
Greater reliability was achieved through over-design, redundancy, and extensive test programs, resulting in larger and more expensive satellites.
Maximizing the performance and longevity of each space vehicle was the only way to achieve cost benefit. These capital assets (large, expensive, rigorous design, but highly reliable satellites with long lifespans) emerged as the dominating design for operational missions.
The divergence of spacecraft development into a capital asset-focused market created a gap in space access that drove the initiation of launch programs such as the Evolved Expendable Launch Vehicle (EELV) and smaller, dedicated launch vehicle designs, necessary to upgrade and modernize access to space. An industry discussion regarding smallsats versus capital assets and their roles in operational satellite utilizations continues to this day.
During the 1990’s, the space industry adopted a “faster, better, cheaper” approach toward satellite development that favored smallsats. Unfortunately, the reality was that satellites could not possess all three of these qualities.
In most cases, a satellite that was faster and better was extremely expensive — one that was quickly and less expensively made was inferior to the quality of capital assets. Additionally, many smallsat developers made unsubstantiated claims that these spacecraft could do everything capital assets were designed to accomplish.
In reality, smaller, less-capable satellites could only accomplish similar functional and performance of their larger cousins through multi-vehicle configurations which, in turn, required better on-board systems and relative navigation technologies that were just starting to
be developed.
be developed.
In hindsight, this mentality actually set the market back a number of years due to some
heralded smallsat failures that did not achieve the cost savings nor mission assurance necessary to achieve operational success.
Though there was hope that smallsats could overtake Capital Assets in popularity during the 1990’s, the inertia of the satellite market remained against commercialization, fueled by government bureaucracy and industry entrenchment.
Smallsat Industry Trends and Enablers
Arguably, the “Smallsat Revolution” began 20 years ago, when cubesat standards were originally defined by Cal Poly, located in San Luis Obispo, and Stanford University in 1999.
The development of cubesats effectively allowed state and non-state actors cost-effective and reliable access to space. They also forced designers and developers to innovate in order to maximize functional and performance attributes within the constraints of the cubesat form factors.
Not unlike many industries’ revolutions (automotive, aviation, computer, etc.), common standards across the smallsat industry became necessary to facilitate new technologies, innovation, and market growth.
Modular Open System Architecture (MOSA) standards began to emerge at the same time as the cubesat standards, with the goal of ensuring interoperability among physical (mechanical and electrical) and software (protocols, etc.) interfaces.
MOSA has been quietly evolving over the last decade, acutely synchronized with the smallsat utilization trend from technology demonstration missions to operational systems and constellations.
Open systems and emerging open-source hardware and software solutions are providing the infrastructure, products, and services necessary for interoperability and integrated mission capabilities, driving today’s smallsat revolution. Standards and open system interfaces allow rapid and responsive systems to be designed and deployed to support government and commercial activities.
These constructs have also supported the development of a robust, worldwide 2nd and 3rd-tier supplier base. This is demonstrated in the Top-1000 NewSpace Companies annual report published by NewSpace People.
Broad commercial growth has also been accompanied by related, low-cost Science, Technology, Engineering and Math (STEM) outreach tools for workforce development. Economic prosperities of the communities built around these initiatives are important by-products of the smallsat revolution.
A prime example of this is the recent boom in aerospace economic drivers along the Front Range region of the Rocky Mountains in Colorado. “Aerospace Alley,” as it is known, consists of more than 200 companies providing hardware and software components, products, and services for the satellite marketplace.
The majority of these companies are small businesses, such as Oakman Aerospace, Inc. (OAI), focused around technology enablers. There are countless of additional, similar examples of these clusters and innovation centers around the world.
OAI plays an important role in this new era of the smallsat revolution, specializing in rapid and responsive MOSA space systems design
and development.
and development.
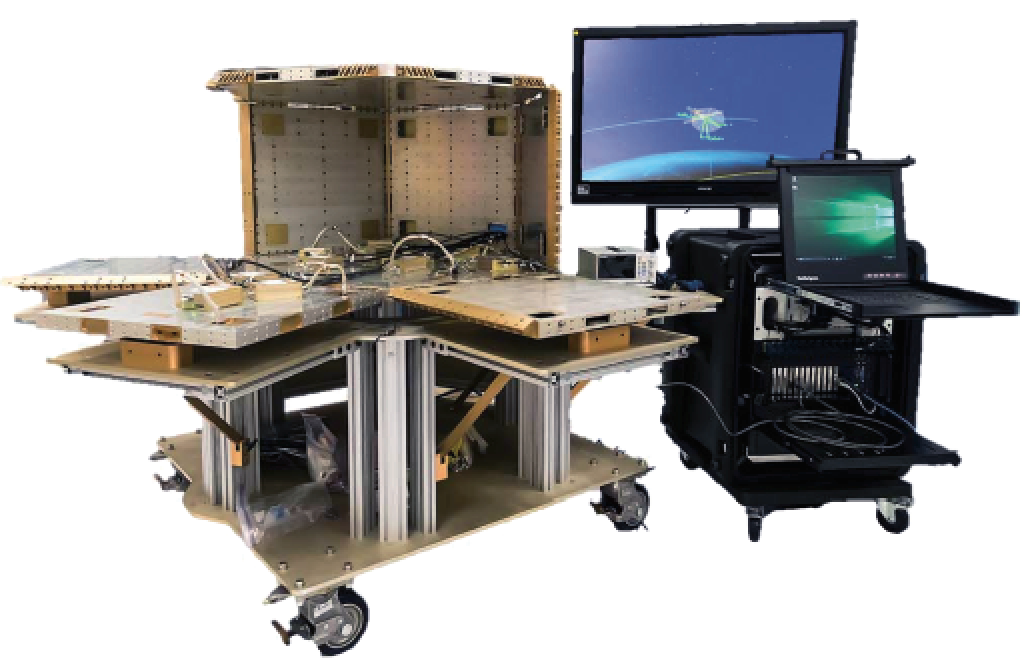
The company’s tagline, “Enabling Your Journey Through Space,” epitomizes the firm’s mission to provide technical capabilities to both emerging and mature customers requiring access to and use of space assets.
OAI is dedicated to providing cutting-edge products and services related to space system architectures, spacecraft design and development, as well as mission payload and data distribution services.
Pictured above: Oakman Aerospace’ Advanced Configurable Open-system Research Network (ACORN).
As a global provider of space-based solutions, OAI offers complete mission life-cycle capabilities for our customers, teammates, and stakeholders.
As the company’s inception in 2012, the OAI Team has established themselves as leaders and contributors to the communities of Spacecraft Standards and Open System Architectures.
Beginning with membership on the Integrated Systems Engineering Team (ISET), OAI personnel helped to define and develop the Operationally Responsive Space Bus Standards that were the pre-cursors to MOSA and are reflected today in our Advanced Configurable Open-system Research Network (ACORN) products and services.
The ACORN framework is a foundational capability to support spacecraft design, integration, test, and operations for a variety of missions and integration efforts. Implementing MOSA, ACORN provides a scalable and expandable, rapidly reconfigurable, closed-loop, end-to-end space systems architecture modeling and simulation environment which provides open, standardized interfaces for segments, elements, subsystems, and components. From OAI’s perspective, the trend of MOSA constructs will be a primary driver/enabler for the next generation of smallsat missions.
Organizations, such as the International Standards Organization (ISO) and the Consultative Committee for Space Data Systems (CCSDS), are the common standard providers and the compliance baseline for the international aerospace market.
Accepted standards ensure that future innovations, fueled by new technologies within the Smallsat industry continue to support interoperability while promoting commercial viability. Electronic Data Sheet (EDS) standardization is a prime example of the next steps in facilitating the interoperability needed to drive the revolution.
Advanced mission and system development in the space industry requires reliable, consistent, and standardized EDS as the foundation for interoperability and increased mission assurance; especially when large space architectures involve multi-national government, academic, and industry participants. EDS standardization is not only necessary for spacecraft design and development activities, but for facilitating the latest discussion topics in the future of space-based capabilities: Machine Learning (ML) and
Artificial Intelligence (AI)
ML/AI, as evident in the Aerospace industry’s vast R&D efforts in these technology realms, is widely considered the next great enabler for smallsats to further achieve space-based effects delivery and ensure continued market growth.
Significant advances in on-board processing technology over the last ten years have made neural networks and heterogeneous computing clusters possible.
Previously, considerable challenges in neural network design included the time and computational power required to train the network. Recent developments in hardware technologies, such as Graphics Processor Units (GPUs), have reduced time and power requirements substantially.
These GPUs are specifically built to handle large-scale matrix multiplication, a very common mathematical operation found in deep learning networks, making them a key capability for efficient neural network training.
Additionally, many machine learning and neural network frameworks have integrated support for GPU processing, ensuring it is easier for neural network developers to use the advanced capabilities of GPUs when developing and training networks. One convenient property of most neural network models is that the computational heavy lifting is front-loaded during the training and prediction phases.
Employing the increased power and processing speed of GPUs into heterogeneous computing clusters results in a robust deep learning training workflow. These scalable models execute a great amount of computations in parallel, enabling the development of influential neural networks that are a critical component for engaging AI in space computing. There is no doubt, ML/AI will continue to accelerate the Smallsat Revolution.
The Future of Smallsats
In assessing the near-term development of emerging technologies as they relate to smallsats, we can predict that the future of the smallsat industry will be truly revolutionary.
Large constellations of operational smallsats are envisioned to provide the world with instant access to data and satellite services. This vision is commonly known as Space Dial Tone and it will operate similar to today’s mobile phone devices, automatically connecting to the nearest available cellular tower.
Today, users needing access to space-based data are in the “switchboard” era, scheduling data downlink passes. The future of ground operations dependent on space-based data, whether military or commercial, will no longer require traditional logistics of scheduling overhead satellite passes and mission management to access data.
Multiple constellations will offer consumers options and access to satellites overhead, while the emerging standards ensure the interoperability of ground-based tools (such as cell phones) to the on-orbit satellites.
Space Dial Tone can be an enabler of multi-domain operations — seamless command, control, data routing and decision-making enterprise across the major domains: Space, Air, Maritime, Land, and Cyber. Currently, multi-domain operations are inhibited by data latency within the decision making process.
This has significant effects in decision confidence and decision execution, negatively affecting the end user. Data latency largely stems from the fact that each of the domains are plagued with interoperability issues, causing delays in data transfer and reliability. The future of the United States military, its Allied Partners, and even commercial industry, will rely on seamless, multi-domain operational solutions.
Data latency within the domains will be mitigated through operational Space Dial Tone constellations as terrestrial users (no matter location: contested theater, remote, desolate, or highly populated) will be able to instantaneously connect to other users or data centers and acquire necessary information. Space Dial Tone’s success is dependent on the enabling constructs of MOSA and interoperability discussed earlier.
Once effectively established, maintaining Space Dial Tone constellations will force continued innovation. Composable and modular spacecraft are necessary in order to scale with continuous technology advancement, especially in the niche market of high performance sensors, effectors, and mission payloads.
Rapid, streamlined manufacturing capabilities will also play a crucial part in future innovation, highlighted by developments being made in the additive manufacturing sector.
Contrary to launch providers of the 1970’s and 1980’s, today’s commercial launch providers are in a competitive market which expects cost-effective, straightforward access to space. Both a scalable spacecraft bus and access to space are necessary for rapid on-orbit replacement within these constellations.
Ongoing innovations and technological advancements, sustained by the key enablers of MOSA and interoperability, will continue to drive the smallsat revolution forward.
During this important time in the smallsat industry, the road to solving future technical challenges promises to be an exciting journey.
At Oakman Aerospace, the company will continue to remain at the forefront of the smallsat revolution — working tirelessly to “Enable Your Journey Through Space.”
Oakman Aerospace, Inc. was co-founded by Stanley Oakman Kennedy, Jr., Maureen S. O’Brien, and Stanley Oakman Kennedy, Sr. in July 2012. While deciding on the name of the new company, they wanted to honor their family heritage and principles that had provided the opportunity and financial abilities to start the new company. The name “Oakman” has been part of the Kennedy family’s Scottish traditions for over 100 years solely in the United States and many hundreds of years throughout the world.